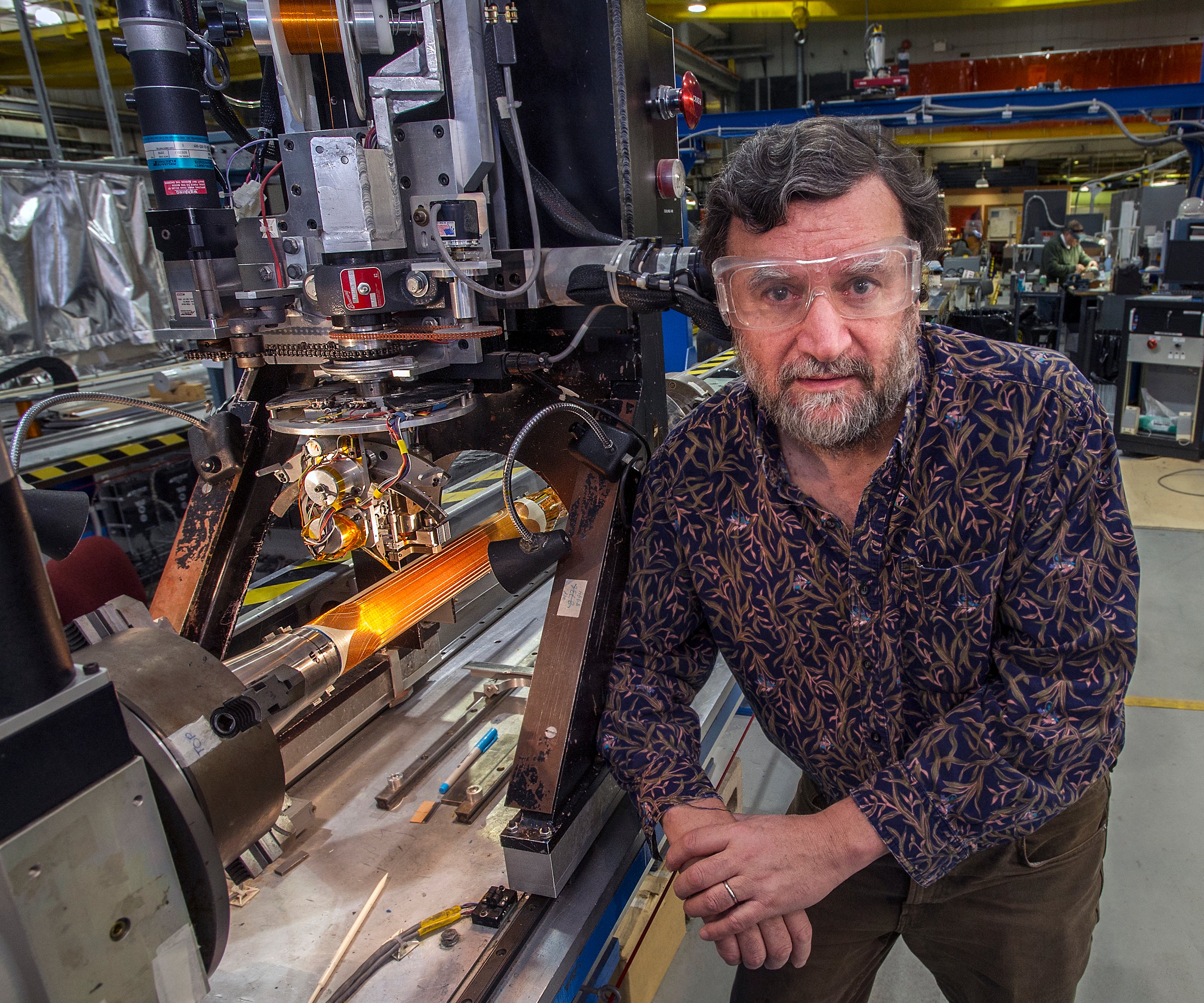
March 7, 2014 — Accelerator physicists are natural-born problem solvers, finding ever more powerful ways to generate and steer particle beams for research into the mysteries of physics, materials and matter. And from the very beginning, this field born at the dawn of the atomic age has actively sought ways to apply advanced technologies to tackle more practical problems. At the top of the list—even in those early days— was taking aim at cancer.
Using beams of accelerated protons or heavier ions such as carbon, oncologists can deliver cell-killing energy to precisely targeted tumors—and do so without causing extensive damage to surrounding healthy tissue. That eliminates the major drawback of conventional radiation therapy using x-rays.
“This is cancer care aimed at curing cancer, not just treating it,” said Ken Peach, a physicist and professor at the Particle Therapy Cancer Research Institute at Oxford University.
Peach was one of six participants in a symposium exploring the latest advances and challenges in this field at the 2014 meeting of the American Association for the Advancement of Science in Chicago, Feb. 16. The session, “Targeting Tumors: Ion Beam Accelerators Take Aim at Cancer,” was organized by the U.S. Department of Energy’s (DOE’s) Brookhaven National Laboratory, an active partner in an effort to build a prototype carbon-ion accelerator for medical research and therapy. Brookhaven Lab is also currently the only place in the U.S. where scientists can conduct fundamental radiobiological studies of how beams of ions heavier than protons, such as carbon ions, affect cells and DNA.
“We could cure a very high percentage of tumors if we could give sufficiently high doses of radiation, but we can’t because of the damage to healthy tissue,” said radiation biologist Kathryn Held of Harvard Medical School and Massachusetts General Hospital during her presentation. “That’s the advantage of particles. We can tailor the dose to the tumor and limit the amount of damage in the critical surrounding normal tissues.”
Yet despite the promise of this approach and the emergence of encouraging clinical results from carbon treatment facilities in Asia and Europe, there are currently no carbon therapy centers operating in the United States.
Participants in the Brookhaven-organized session agreed: That situation has to change—especially since the very idea of particle therapy was born in the United States. Much of the initial radiation biology and clinical work was done at DOE’s Lawrence Berkeley National Laboratory, and U.S. physicists have been pioneers in the field. The session explored this rich history, the rationale and technology for proton and carbon-ion therapy, and how a carbon-ion treatment facility might be built in the United States.
The session kicked off with an introduction from moderator James Deye, program director for the Division of Cancer Treatment and Diagnosis within the Radiation Research Program of the National Cancer Institute. “We’ll hear about something old, something new, and a lot of things borrowed,” he said, suggesting that a “marriage” joining scientists from diverse disciplines might be the key to realizing the promise of particle therapy.
Stephen Peggs, an accelerator physicist at Brookhaven Lab and adjunct professor at Stony Brook University, recapped the birth of the idea of particle therapy and the field of accelerator science just after World War II—both coinciding with the formation of the U.S. national laboratories, now run by DOE.
“When Harvard physicist Robert Wilson, who later became the first director of Fermilab [Fermi National Accelerator Laboratory], was asked to explore the potential dangers of proton particle radiation, he flipped the problem on its head and described how proton beams might be extremely useful—as effective killers of cancer cells,” he said.
As Peggs explained, the reason is simple: Unlike conventional x-rays, which deposit energy—and cause damage—all along their path as they travel through healthy tissue en route to a tumor (and beyond it), protons and other ions deposit most of their energy where the beam stops. Using magnets, accelerators can steer these charged particles left, right, up, and down and vary the energy of the beam to precisely place the cell-killing energy right where it’s needed: in the tumor.
The first implementation of particle therapy used helium and other ions generated by a machine built for fundamental physics research, the Bevatron accelerator at Berkeley Lab. Those pioneering spin-off studies “established a foundation for all subsequent ion therapy,” Peggs said. “It was also a ground-breaking and serendipitous demonstration of the transfer of emerging technology from DOE to medicine.”
As accelerators for physics research grew in size, pioneering experiments in particle therapy continued, operating “parasitically” at physics research facilities. The first accelerator built for hospital-based proton therapy was completed with the help of DOE scientists at Fermilab in 1990. But even before that machine left Illinois for Loma Linda University Medical Center in California, said Peggs, who was at Fermilab at the time, physicists were thinking about how it could be made better. The mantra of making machines smaller, faster, cheaper—and capable of accelerating more kinds of ions—has driven the field since then.
Advances in magnet technology, including compact superconducting magnets and beam-delivery systems developed at Brookhaven Lab, hold great promise for new machines. As a principal investigator in a Cooperative Research and Development Agreement (CRADA) contract between Brookhaven Lab and Best Medical International, Peggs is working to incorporate these technologies in a prototype ‘ion Rapid Cycling Medical Synchrotron’ (iRCMS) capable of delivering protons and/or carbon ions for radiobiology research and for treating patients.
The benefits of using charged particles heavier than protons stem not only from their physical properties—they stop and deposit their energy over an even smaller and better targeted tumor volume than protons—but also a range of biological advantages they have over x-rays, as Kathryn Held elaborated in her talk.
Compared with x-ray photons, “carbon ions are much more effective at killing tumor cells,” she said. “They put a huge hole through DNA compared to the small pinprick caused by x-rays, which causes clustered or complex DNA damage that is less accurately repaired between treatments—less repaired, period—and thus more lethal [to the tumor].”
In addition, she said, many human tumors develop regions of hypoxia because the tumor grows faster than the blood supply. “These hypoxic cells are resistant to radiation damage with x-ray photons, but there is less resistance when heavy ions are used,” she said.
Held highlighted how important it will be to conduct further research to explore, for example, which heavy ions are best and how treatment fractions should be delivered. Studies suggest that use of a smaller number of very high dose fractions, called hypofractionation, might be more effective. That would be “an advantage economically and an advantage to patients…but we need more basic biological studies to really understand these effects,” she said.
There are very few places to conduct this research, Held pointed out. One is a facility built by NASA at Brookhaven Lab to explore the effects of space radiation on cells and DNA. The goal of the NASA Space Radiation Laboratory (NSRL) is to fully understand risks and design protections for future astronauts. But much of the research is relevant to understanding the mechanisms and basic radiobiological responses that can apply to the treatment of cancer.
Held conducts research at NSRL, which has been funded by NASA and more recently by NCI, but additional facilities and funding are needed for research specifically aimed at understanding the radiobiological effects of heavier ions for potential cancer therapies, she emphasized.
Hak Choy is a radiation oncologist and chair in the department of radiation oncology at the University of Texas Southwestern Medical Center. He presented data on the benefits of particle therapy, including improved outcomes and reduced side effects when compared with conventional radiation, particularly for treating tumors in sensitive areas such as the brain and spine and in children.
“When you can target the tumor and spare critical tissue you get fewer side effects,” he said. For example, when treating brain tumors with protons, doctors can spare the nerve going to the eye or the cochlea to preserve vision and hearing. Data also show that children with brain tumors treated with protons have better scores on math and spelling tests than children treated with x-rays, he said.
Data from Japan and Europe suggest that carbon ions could be three or four times more biologically potent than protons, Choy said. He presented survival statistics for certain types of cancers where carbon therapy surpassed protons, and was even better than surgery for one type of salivary gland cancer.
“And carbon therapy is noninvasive,” Choy emphasized.
In one study, it appeared carbon therapy combined with chemotherapy boosted the two-year survival rate to 54 percent for pancreatic cancer. That’s nearly double the survival rate for any other form of therapy.
But all these data come from comparisons between different treatment centers where other variables might influence results. In order to make direct comparisons between different treatment methods, you need randomized clinical trials, Choy explained. To conduct such trials you need a comprehensive hospital-based facility, and the cost estimates for building one are around $200-300 million.
To kick off the discussion that followed the presentations, Deye noted that NCI recently announced a funding opportunity to help establish a research agenda for a carbon therapy facility, should one be built in the United States.
“NCI doesn’t do construction, but we do research,” he said, noting that NCI might fund research if a facility is built, or possibly provide support for clinical trials at some of the existing facilities in Asia or Europe.
As Ken Peach summed up, “There is technology available for carbon ion therapy today, there is strong radiobiological evidence for its effectiveness, and there is strong clinical evidence for its effectiveness, but there is a huge amount of work still to be done.
“New clinical facilities are desperately needed worldwide and particularly in the U.S., which was a pioneering leader in this field and has, frankly, fallen behind.”
That statement ignited a lively question and answer session concerning who would pay for such a facility and how those who put up the money could get the needed return on their investment.
Making a comparison with cancer drug development, NCI’s Deye said, “The government doesn’t fund late-stage drug development in this country. That’s funded by big pharma. Maybe we need a comparable industrial investment [to push this technology forward].”
For determining how those investments would be repaid, DOE’s Colby said, “Having a first center in place to demonstrate a business model is very important.”
As Peach pointed out, “If you amortize the cost over the lifetime of the machine the cost per patient is not all that much higher than for conventional therapy.”
Since it may be extremely difficult to convince anyone to make the initial investment, a more realistic way to start may be to build the smaller prototype machine envisioned by the partnership between Brookhaven Lab and Best Medical.
“With a prototype machine,” said Brookhaven’s Peggs, “we can test technologies, fix inevitable glitches, and reduce risks for future clinical facilities” while conducting the needed radiobiological and clinical research.
Choy agreed that prototypes might be the place to start. “If the patients understand the potential benefits of this they will come knocking at the door,” he said. “The big question is to look at what is the optimal design. You don’t want to make the huge investment until you know. We have to build prototypes of different designs to figure that out.”
“One of the really encouraging things,” said Peach, “is that there is this enormous will from the scientists across the different disciplines to try to increase the efficacy of this promising therapy and make it more widely available.”
For more information: www.bnl.gov